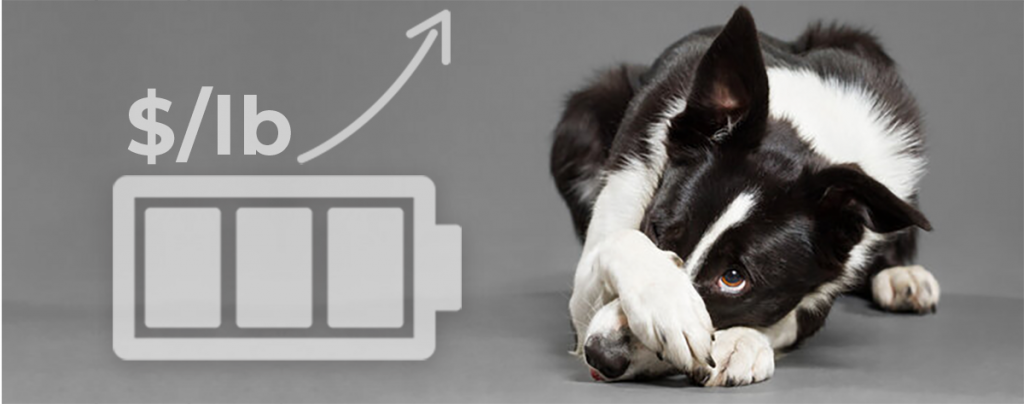
Sales for electric vehicles, consumer electronics and stationary storage are expected to increase lithium-ion demand by double in 2025 and quadruple by 2030. That will create a LOT of spent batteries. Lithium-ion battery recycling is not well developed. Despite the eminent need for recycling, the economics are not financially compelling. The purpose of this article is to discuss these challenges and to share recent recycling costs.
Complicated and Time Consuming
Lithium-ion batteries have a wide variety of materials in each cell. The active materials are in the form of powder that are coated onto metal foils. These different materials must be separated from each other during recycling. Some large format lithium-ion manufacturers encase the cells in epoxy, making deconstruction extremely difficult. In addition, a lithium-ion pack is likely to contain 100 or more individual cells. This makes recycling a costly and complicated process.
Critical Metals
Cobalt, nickel and lithium have been identified by the Biden administration as “critical metals.”[1] Cobalt has driven the business case for recycling, but in the future, the value of the reclaimed nickel and lithium may also help. Unfortunately, the amount of these critical metals represents a small fraction of the total battery weight (low single digit percent). Battery manufacturers require critical metals to have the highest purity, this makes the economics of recycling even more difficult.
Diminishing Cobalt Content
Manufacturers have been systematically reducing their dependency on cobalt. As the cobalt content diminishes, so does the immediate motivation to recycle. Cobalt creates substantial supply chain risk for battery manufacturers due to its price volatility (prices have ranged from USD $10-$42/ton). Additionally, cobalt mining has issues with human rights, including child labor. The Democratic Republic of Congo (DRC) is by far the world’s largest producer of cobalt, accounting for roughly 60 percent of global production. Avoiding DRC sourced cobalt creates further pressure on prices.
Following the commercial success of equally blended NMC[2] (⅓ nickel, ⅓ manganese, ⅓ cobalt – also abbreviated as NMC 111), NMC cathodes have migrated to a lower cobalt ratio. Many EV and stationary storage battery makers are now using NMC 811 (cathode composition with 80% nickel, 10% manganese, and 10% cobalt). Lithium Iron Phosphate (LFP) batteries have been gaining market share due to their low cost (no cobalt content).
These trends underscore one of the fundamental challenges that will complicate the future of the recycling landscape. Without cobalt, there may be little financial incentive to recycle batteries or to invest in recycling technologies (without subsidies or grants).
U.S. Legislation is Lagging
The European Union (EU) has implemented a directive for collection and recycling of batteries. The EU Battery Directive (2006/66/EC) regulates the manufacturing, disposal and accumulators of batteries in the EU to minimize the negative impact on the environment. Most notably:
- Battery producers or third parties acting on their behalf cannot refuse to take back waste batteries.
- All collected batteries must be recycled.
- Batteries cannot be disposed of in landfills or by incineration.
- Recycling processes must achieve a minimum efficiency of 65% for lead-acid batteries, 75% for nickel-cadmium batteries and 50% for other batteries.
The U.S. pales in comparison. In 2017, the Trump administration introduced EO 13817 – A Federal Strategy to Ensure Secure and Reliable Supplies of Critical Minerals.[3] The EO cited the national dependency on foreign sources for certain mineral commodities (lithium and cobalt). The EO called for the following actions:
- Increasing private-sector domestic exploration, production, recycling, and reprocessing of critical minerals, and support to identify alternatives
- Increasing activity at all levels of the supply chain to expedite exploration, production, processing, reprocessing, recycling, and domestic refining of critical minerals
In response to the EO, the Department of Energy Vehicle Technologies Office (VOT) initiated three areas of R&D:[4]
- Supporting laboratory, university, and industry research to develop low-cobalt (or no cobalt) active cathode materials for next-generation lithium-ion batteries.
- Establishing the ReCell Lithium Battery Recycling R&D Center focused on cost effective recycling processes to recover lithium battery critical materials.[5]
- Launching a Lithium-Ion Battery Recycling Prize[6] to incent American entrepreneurs to find innovative solutions to solve current challenges associated with collecting, storing, and transporting discarded lithium-ion batteries for eventual recycling.
Unfortunately, despite all the climate friendly ambitions our country has postulated, our plan for domestic recycling is nowhere close to maturity.
Recycling Costs Have Actually Increased
Prior to 2018, numerous U.S. companies claimed to “recycle” lithium-ion batteries, but in actuality they were shipping the batteries to China (similar to plastics). For over a decade, recyclables and scrap materials have been one of the country’s largest exports to China. In 2018, China enacted the National Sword policy restricting plastic waste imports to protect their environment and to develop their own domestic recycling capacity. In addition to the bans, China reduced the number of import licenses, meaning that fewer businesses could import waste.[7] In response, many recyclers moved their operations from China to other countries in Asia. Exports from the U.S. to Thailand jumped almost 7,000 percent in one year, while Malaysia’s went up several hundred percent.[8] The actual amount of U.S. plastic waste that ends in countries with high waste mismanagement may be even higher because the U.S. exports millions of kgs of plastic waste to countries like Canada and South Korea who may re-export U.S. plastic waste to other countries.
Current Recycling Costs
Fractal received a quote from a recycling company in Q2/2021 that costs $1.00/lb to collect and accept batteries from a project site. This includes the cost of pick up and transport by a qualified Universal Waste Handler. But this does not include the cost of packaging the batteries onto pallets (about $0.50/lb). It is unknown what happens to the batteries once they are accepted by the recycling company. Sadly, they are most likely put in a landfill.
Non-Lithium Batteries
Quotes for Sodium Sulfur ($1.70/lb) and Zinc Air ($1.85/lb) batteries were also received. Keep in mind that Fractal only received quotes from two companies. Other recylers may have better pricing, volume discounts or corporate partnerships.
Impact of Energy Density
Since we know that recycling costs are a function of weight, let us exam how energy density impacts recycling costs. Note: The number of enclosures, modules and weights will vary across vendors, but this is an example of density variations across battery chemistries. Fractal has intentionally omitted the total cost to preserve your sanity.
Chemistry | Lithium NMC | Lithium LFP | Zinc Air |
Power/Energy | 10 MW / 40 MWh | 10 MW / 40 MWh | 10 MW / 40 MWh |
Footprint | 10 x 40ft Containers 442 modules / container | 20 x 40ft Containers 256 modules / container | 320 x 25ft Containers 144 modules / container |
Weight | 148.77 lbs / module Total Weight: 657,563 lbs | 198.42 lbs / module Total Weight: 1,015,910 lbs | 215 lbs / module Total Weight: 9,907,200 lbs |
Fractal Energy Storage Consultants is a consulting and OE firm that specializes in energy storage and hybrid systems. More information at https://www.energystorageconsultants.com
[1] https://abcnews.go.com/Politics/biden-sign-executive-order-aimed-securing-critical-us/story?id=76077342
[2] Lithium Nickel Manganese Cobalt Oxide
[3] https://www.federalregister.gov/documents/2017/12/26/2017-27899/a-federal-strategy-to-ensure-secure-and-reliable-supplies-of-critical-minerals
[4] https://www.energy.gov/sites/prod/files/2019/07/f64/112306-battery-recycling-brochure-June-2019%202-web150.pdf
[5] https://www.anl.gov/article/doe-launches-its-first-lithiumion-battery-recycling-rd-center-recell
[6] The $5.5-million, three-phased Lithium-Ion Battery Recycling Prize was announced by Secretary of Energy Rick Perry in January 2019. https://americanmadechallenges.org/batteryrecycling/
[7] https://www.centerforecotechnology.org/what-is-the-national-sword/
[8] https://news.sky.com/story/malaysia-sends-plastic-waste-back-to-uk-insisting-it-is-not-worlds-rubbish-dump-11913156
read more
Recent Comments